Making sense of scents: 3D videos reveal how the nose detects odor combinations
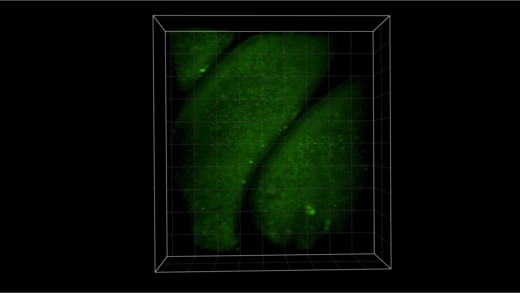
Every moment of the day we are surrounded by smells. Odors can bring back memories, or quickly warn us that food has gone bad. But how does our brain identify so many different odors? And how easily can we untangle the ingredients of a mixture of odors? In a new study in mice published today in Science, Columbia scientists have taken an important step toward answering these questions, and the secret lies inside the nose. "From garbage to cologne, the scents we encounter every day are comprised of hundreds or even thousands of individual odors," said Stuart Firestein, PhD, a Columbia professor of biological sciences and the co-senior author of today's study. "Your morning cup of coffee can contain more than 800 different types of odor molecules. Although much work has been done to understand how the nose and brain work together to identify individual odors, scientists have long struggled to explain how this system works when multiple odors are mixed together." Using a cutting-edge 3D imaging method called SCAPE microscopy, the Columbia team monitored how thousands of different cells in the nose of a mouse responded to different odors -- and mixtures of those odors. They found that the information that the nose sends to the brain about a mixture of scents is more than just the sum of its parts. The cells in the nose that detect smells each have one of a wide range of different sensors, or receptors; humans, for example have up to 400 different types of these receptors. For a pure, single odor, only the cells whose receptors are sensitive to that odor will become active, sending a code to the brain that it can identify as that odor. But for more complex mixtures of odors, this code would become increasingly complex to interpret. The researchers expected to see that the cells activated by mixtures of odors would be equivalent to adding together responses to individual odors. In fact, they found that in some cases an odor can actually turn off a cell's response to another odor in a mixture; in other cases, a first odor could amplify a cell's response to a second odor. Although we often perceive one odor dominating another, it was previously assumed that this processing occurred in the brain. These results show that signals being sent to the brain get shaped by these interactions within the nose. The team's data challenged the traditional view that the brain makes sense of a mixture of scents by figuring out all of the individual components. It confirmed what perfumers have long known: combining different scents can create a certain experience on its own, essentially becoming an entirely new scent that can provide a completely different experience. "We were excited to find that these changes in the code happened in the nose, before signals even get to the brain," said Lu Xu, a doctoral candidate in the Firestein lab and the co-first author of today's study. "We think that these effects could help us to detect and identify a much larger range of odors and mixtures than a simple additive code could convey." To reveal these inner workings of the olfactory system, the researchers harnessed the power of SCAPE microscopy, a technique developed by Elizabeth Hillman, PhD, a Zuckerman Institute principal investigator and the co-senior author of today's Science paper. SCAPE microscopy creates high-speed, 3D images of living tissues in real time. It sweeps an angled sheet of light back and forth to create high-speed 3D movies of living cells and tissues in action. The Firestein and Hillman labs customized SCAPE to illuminate and view tissues in the noses of mice. The researchers examined neuronal cells within the animals' noses that were labeled with fluorescence that flashed under the microscope when these cells were activated. They then exposed the animals' nasal tissues to a range of different scent combinations: one with a woody bouquet, and the other a mix of almond, floral and citrus scents. "SCAPE allowed us to simultaneously analyze the activity in any of the tens of thousands of single cells over long periods of time," said Wenze Li, PhD, a postdoctoral research scientist in the Hillman lab and the paper's co-first author. "Using conventional microscopes, we could only image a few hundred cells in a thin layer for a short time. SCAPE made it possible to image many more cells within the intact 3D nasal structures without damaging the tissue. This enabled us to track each cell's response over time to a long series of different odor combinations." To process the immense amount of data collected -- more than 300 gigabytes per tissue sample -- the team had to build their own powerful data processing server, and worked with algorithms developed by Columbia's Department of Statistics and the Simons Foundation. For almost twenty years, scent experts have known that certain smells can mask or even enhance others. With today's study, the researchers uncovered a potential mechanism for this phenomenon. "Our results showed that scent molecules can both activate and deactivate receptors, masking other scents not by overpowering them, but by changing the way cells respond to them," said Dr. Hillman, who is professor of biomedical engineering at Columbia School of Engineering and Applied Science. "These findings could actually be very useful, for example to make better air fresheners that actually block out any unwanted smells." "These results are also exciting because we didn't expect that this kind of receptor could be enhanced or suppressed in this way," added Dr. Firestein. "Being able to change the way a receptor responds to one substance is very important for drug development. Our studies in the nose actually shed new light on possible ways to modulate the response of other cell types that might be involved in disease." "This study was a true marriage of the expertise of two different labs, with state-of-the-art microscopy technology and big-data analysis," added Dr. Hillman, who is also a professor of radiology at Columbia's Vagelos College of Physicians and Surgeons. Dr. Hillman credits funding and encouragement from the NIH BRAIN Initiative for making this parallel science and technology work possible. "We discovered something that was almost impossible to see before, because we had a new way to see it." Widespread receptor driven modulation in peripheral olfactory coding Lu Xu, Wenze Li, Venkatakaushik Voleti, Dong-Jing Zou, Elizabeth M. C. Hillman, Stuart Firestein Science (2020) DOI: 10.1126/science.aaz5390 Contact information: Stuart Firestein Columbia professor of biological sciences sjf24@columbia.edu Phone: (212) 854-4531 Firestein Lab Elizabeth Hillman Columbia professor of biomedical engineering; Zuckerman Institute principal investigator elizabeth.hillman@columbia.edu Phone: 212-853-1098 Hillman Lab The Zuckerman Institute at Columbia University
Learn more about the Nanotechnology World Association (NWA):
Comments