Entanglement inside proton ‘X-rayed’ with quantum information tools
- Mateo Cardinal
- Feb 7
- 5 min read
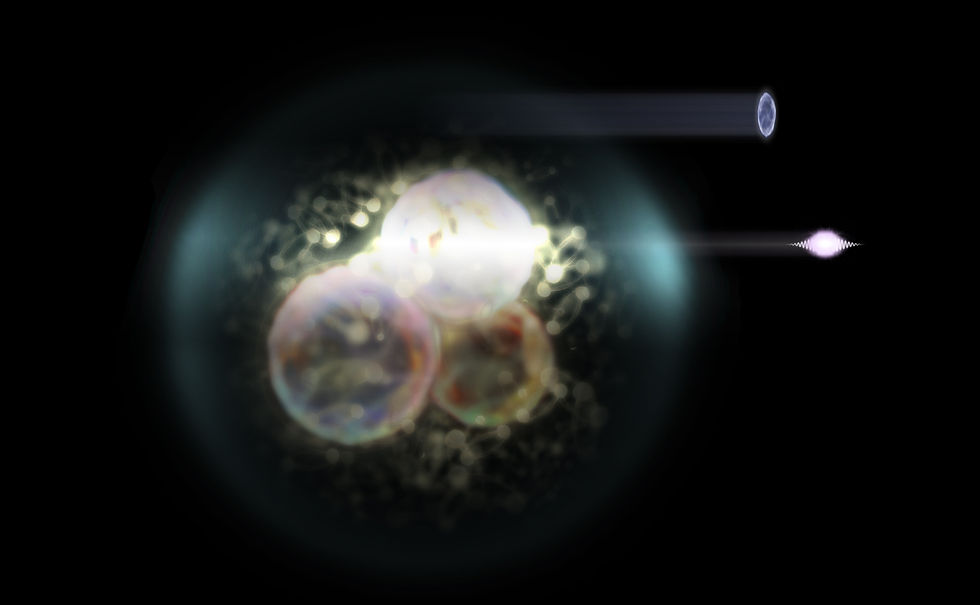
In recent years, there have been attempts to describe the inside of a proton using quantum information tools, with partial success. It has been discovered that quantum entanglement in the proton is maximal, and that its main sources are constantly ‘boiling’ seas of virtual gluons and quarks. In the article just published, this new formalism has already become so universal that it correctly reproduces, for the first time, all currently available experimental data.
It is difficult to imagine a world more dynamic and at the same time more inaccessible than the inside of a proton. The complex interactions of its constituent quarks, gluons and the constantly ‘churning’ sea of virtual particles can now be coherently described thanks to the skilful use of the tools of quantum information theory and the phenomenon of quantum entanglement. The new, more than hitherto universal formalism has made it possible, for the first time, to explain data from all available measurements related to the scattering of secondary particles produced during deeply inelastic collisions between electrons and protons. The team responsible for the achievement include theorists from Brookhaven National Laboratory (BNL) and Stony Brook University (SBU) in New York, Mexico's Universidad de las Americas Puebla (UDLAP) and the Institute of Nuclear Physics of the Polish Academy of Sciences (IFJ PAN) in Cracow.
“If we want to learn about the phenomena taking place inside a proton, we first have to get there somehow. At present, collisions between protons and electrons are the best way to do this, because the latter are not only much smaller in size than protons, but, above all, they are elementary particles, so we have a guarantee that they themselves will not decay into anything else,” says Prof. Krzysztof Kutak (IFJ PAN), one of the authors of the article published in Reports on Progress in Physics.
The proton is not an elementary particle. In the simplest terms, it is assumed to consist of three valence quarks (two up and one down) ‘stuck together’ by means of gluons, i.e. particles carrying strong interactions. These interactions are so powerful that, inside the proton, pairs of virtual quarks and anti-quarks (even as massive as charm) and pairs of virtual gluons (which is possible because these particles are antiparticles to each other) constantly appear and disappear.
In the research described here, the key assumption was that, despite the extremely small size of the proton, the quarks and gluons that make it up – collectively called partons – are quantum entangled. We speak of entanglement between quantum objects when the values of a feature of one object react to its changes in another object, despite the fact that the information about the change has not had time to be transmitted between them by any carrier transported through space.
“In the case of the interior of the proton, entanglement occurs at difficult to imagine distances of one quadrillionth of a metre or less and is a collective feature. As we have shown in our earlier publications, it affects not a few, but all partons in the proton,” says Prof. Martin Hentschinski (UDLAP).
When, in an attempt to explore the maximally entangled interior of a proton, an electron strikes it, an electromagnetic interaction occurs between the two particles, the carrier of which is a photon. In deeply inelastic collisions, the energy of the exchanged photon is so high that the associated electromagnetic wave begins to ‘fit’ inside the proton and ‘see’ details of its internal structure. As a result of the interaction with the photon, the proton can then decay producing numerous secondary particles. Entanglement will manifest itself here in the fact that the number of secondary particles emitted from the part of the proton ‘noticed’ by the photon will determine the number of particles that will be produced as observed hadrons.
“This is how we arrive at the concept of entropy, which is particularly important in the study of highly complex systems and in quantum information. If, thanks to deeply inelastic collisions, we had access to the full entanglement information in the proton, we could speak of an entanglement entropy of zero. However, a photon penetrating the inside of a proton ‘sees’ only part of the proton's interior, the rest remains hidden to it – and this means that the entanglement entropy is nonzero. We therefore have a convenient measure of the amount of entanglement in the proton,” explains Prof. Dmitri Kharzeev (SBU, BNL).
In the paper in question, the international team of physicists proved that on the basis of entanglement entropy, the entropy of hadrons produced in an electron-proton collision can be predicted. As a result, the maximal entanglement of quarks and gluons in a proton manifests itself in the impossibility of determining how many particles will be produced in a particular collision. These predictions have now been verified for all variants of the measurements carried out in 2006-2007 in the H1 experiment at the HERA particle accelerator at the DESY centre in Hamburg, where single protons collided with positrons, the antiparticles of electrons.
“We have been working on entanglement inside the proton for several years now. While we verified our previous theoretical work by confronting measurements from specific measurement sessions, we have now managed to describe all the experimental deep inelastic scattering entropy data within a single universal formalism,” emphasises Dr. Zhoudunming Tu (BNL).
The team of physicists involved in the project anticipates that it is the generalised formalism that will enable easier and more accurate interpretation of measurements from future colliders, such as the Electron-Ion Collider (EIC), which will be launched at Brookhaven Laboratory early next decade. Here, electrons will collide not only with individual protons, but with ions. Combined with new experimental data, the proposed theoretical approach should then help to unravel important problems in modern nuclear physics.
“Today, we have a strong indication that our new formalism taking entanglement entropy into account is not randomly correlated with some particular way of measuring nuclear phenomena, but has a real ability to explain the nature of observed events. We are convinced that by studying entanglement entropy, we will be able to better understand how strong interactions bind quarks and gluons in protons or answer the question of how belonging to a larger atomic nucleus affects the properties of a single proton,” concludes Prof. Kutak. Reference QCD evolution of entanglement entropy
Martin Hentschinski, Dmitri E Kharzeev, Krzysztof Kutak and Zhoudunming Tu https://iopscience.iop.org/article/10.1088/1361-6633/ad910b Institute of Nuclear Physics of the Polish Academy of Sciences (IFJ PAN)
Commenti