Caltech-led team finds new superconducting state
- Mateo Cardinal
- Mar 31
- 3 min read
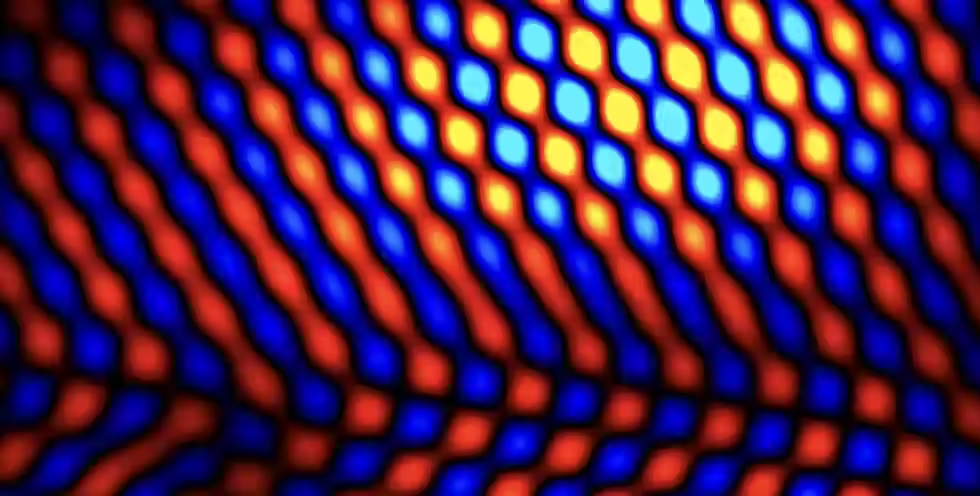
Superconductivity is a quantum physical state in which a metal is able to conduct electricity perfectly without any resistance. In its most familiar application, it enables powerful magnets in MRI machines to create the magnetic fields that allow doctors to see inside our bodies. Thus far, materials can only achieve superconductivity at extremely low temperatures, near absolute zero (a few tens of Kelvin or colder). But physicists dream of superconductive materials that might one day operate at room temperature. Such materials could open entirely new possibilities in areas such as quantum computing, the energy sector, and medical technologies. "Understanding the mechanisms leading to the formation of superconductivity and discovering exotic new superconducting phases is not only one of the most stimulating pursuits in the fundamental study of quantum materials but is also driven by this ultimate dream of achieving room-temperature superconductivity," says Stevan Nadj-Perge, professor of applied physics and materials science at Caltech.
Now a team led by Nadj-Perge that includes Lingyuan Kong, AWS quantum postdoctoral scholar research associate, and other colleagues at Caltech has discovered a new superconducting state—a finding that provides a new piece of the puzzle behind this mysterious but powerful phenomenon. A paper about the work was published on March 19 in the journal Nature.
In normal metals, individual electrons collide with ions as they move across the metal's lattice structure made up of oppositely charged ions. Each collision causes electrons to lose energy, increasing electrical resistance. In superconductors, on the other hand, electrons are weakly attracted to each other and can bind, forming duos called Cooper pairs. As long as the electrons stay within a certain relatively small range of energy levels known as the energy gap, the electrons remain paired and do not lose energy through collisions. Therefore, it is within that relatively small energy gap that superconductivity occurs.
Typically, a superconductor's energy gap is the same at all locations within the material. For example, in a superconducting crystal without impurities, all pieces of the crystal would have the same energy gap.
But beginning in the 1960s, scientists began theorizing that the energy gap in some superconducting materials could modulate in space, meaning the gap could be stronger in some areas and weaker in others. Later, in the 2000s, the idea was further developed with the proposal of what is called the pair density wave (PDW) state, which suggests that a superconducting state could arise in which the energy gap modulates with a long wavelength, where the gap fluctuates between a larger and smaller measurement.
Over the past decade, this concept has garnered significant experimental interest with numerous materials, including iron-based superconductors being explored as potential hosts of a PDW state.
Now, working with extremely thin flakes of an iron-based superconductor, FeTe0.55Se0.45, Nadj-Perge and his colleagues have discovered a modulation of the superconducting gap with the smallest wavelength possible, matching the spacing of atoms in a crystal. They have named it the Cooper-pair density modulation (PDM) state.
"The observed gap modulation, reaching up to 40 percent, represents the strongest reported so far, leading to the clearest experimental evidence to date that gap modulation can exist even at the atomic scale," says Kong, lead author of the new paper.
This unexpected discovery was made possible by the first successful realization of scanning tunneling microscopy experiments of an iron-based superconductor on a specialized device for studying such thin flakes. Such experiments had been hampered for nearly two decades by the presence of severe surface contamination, but the Caltech team, working in the Kavli Nanoscience Institute (KNI), developed a new experimental approach that enabled a sufficiently clean surface for microscopic probes.
Michał Papaj, assistant professor of physics at the University of Houston, and Patrick A. Lee, William & Emma Rogers Professor of Physics at MIT and visiting associate at Caltech, both also authors of the new paper, developed a theoretical model to explain the possible origin of the observed gap modulation. "Their model suggests that this PDM state modulation arises from the breaking of both sublattice symmetry and a unique rotational symmetry specific to thin flakes," Nadj-Perge says. Reference Cooper-pair density modulation state in an iron-based superconductor
Lingyuan Kong, Michał Papaj, Hyunjin Kim, Yiran Zhang, Eli Baum, Hui Li, Kenji Watanabe, Takashi Taniguchi, Genda Gu, Patrick A. Lee & Stevan Nadj-Perge https://www.nature.com/articles/s41586-025-08703-x Caltech
Comments