NIST team supersizes ‘quantum squeezing’ to measure ultrasmall motion
- Marine Le Bouar
- Jun 22, 2019
- 3 min read
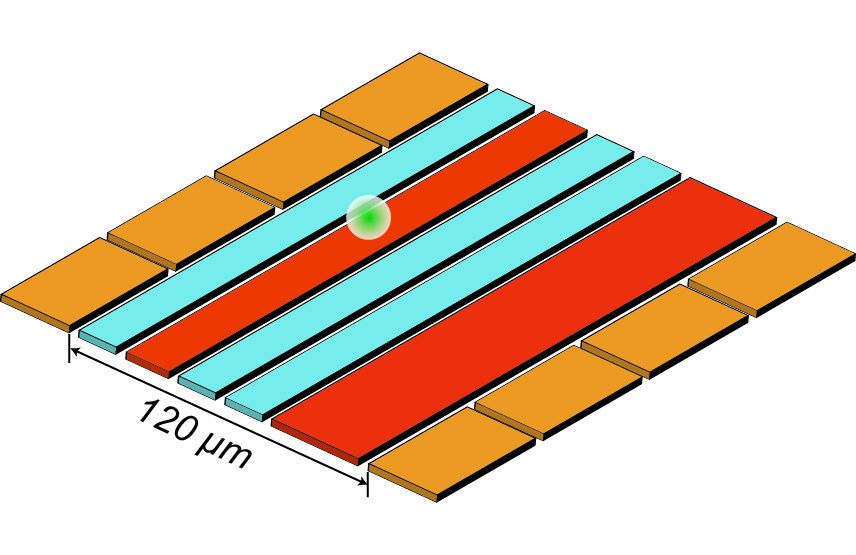
Physicists at the National Institute of Standards and Technology (NIST) have harnessed the phenomenon of “quantum squeezing” to amplify and measure trillionths-of-a-meter motions of a lone trapped magnesium ion (electrically charged atom).
Described in the June 21 issue of Science, NIST’s rapid, reversible squeezing method could enhance sensing of extremely weak electric fields in surface science applications, for example, or detect absorption of very slight amounts of light in devices such as atomic clocks. The technique could also speed up operations in a quantum computer.
“By using squeezing, we can measure with greater sensitivity than could be achieved without quantum effects,” lead author Shaun Burd said.
“We demonstrate one of the highest levels of quantum squeezing ever reported and use it to amplify small mechanical motions,” NIST physicist Daniel Slichter said. “We are 7.3 times more sensitive to these motions than would be possible without the use of this technique.”
Although squeezing an orange might make a juicy mess, quantum squeezing is a very precise process, which moves measurement uncertainty from one place to another.
Imagine you are holding a long balloon, and the air inside it represents uncertainty. Quantum squeezing is like pinching the balloon on one end to push air into the other end. You move uncertainty from a place where you want more precise measurements, to another place, where you can live with less precision, while keeping the total uncertainty of the system the same.
In the case of the magnesium ion, measurements of its motion are normally limited by so-called quantum fluctuations in the ion’s position and momentum, which occur all the time, even when the ion has the lowest possible energy. Squeezing manipulates these fluctuations, for example by pushing uncertainty from the position to the momentum when improved position sensitivity is desired.
In NIST’s method, a single ion is held in space 30 micrometers (millionths of a meter) above a flat sapphire chip covered with gold electrodes used to trap and control the ion. Laser and microwave pulses are applied to calm the ion’s electrons and motion to their lowest-energy states. The motion is then squeezed by wiggling the voltage on certain electrodes at twice the natural frequency of the ion’s back-and-forth motion. This process lasts only a few microseconds.
After the squeezing, a small, oscillating electric field “test signal” is applied to the ion to make it move a little bit in three-dimensional space. To be amplified, this extra motion needs to be “in sync” with the squeezing.
Finally, the squeezing step is repeated, but now with the electrode voltages exactly out of sync with the original squeezing voltages. This out-of-sync squeezing reverses the initial squeezing; however, at the same time it amplifies the small motion caused by the test signal. When this step is complete, the uncertainty in the ion motion is back to its original value, but the back-and-forth motion of the ion is larger than if the test signal had been applied without any of the squeezing steps.
To obtain the results, an oscillating magnetic field is applied to map or encode the ion’s motion onto its electronic “spin” state, which is then measured by shining a laser on the ion and observing whether it fluoresces.
Using a test signal allows the NIST researchers to measure how much amplification their technique provides. In a real sensing application, the test signal would be replaced by the actual signal to be amplified and measured.
The NIST method can amplify and quickly measure ion motions of just 50 picometers (trillionths of a meter), which is about one-tenth the size of the smallest atom (hydrogen) and about one-hundredth the size of the unsqueezed quantum fluctuations.
Even smaller motions can be measured by repeating the experiment more times and averaging the results. The squeezing-based amplification technique allows motions of a given size to be sensed with 53 times fewer measurements than would otherwise be needed.
Squeezing has previously been achieved in a variety of physical systems, including ions, but the NIST result represents one of the largest squeezing-based sensing enhancements ever reported.
NIST’s new squeezing method can boost measurement sensitivity in quantum sensors and could be used to more rapidly create entanglement, which links properties of quantum particles, thus speeding up quantum simulation and quantum computing operations. The methods might also be used to generate exotic motional states. The amplification method is applicable to many other vibrating mechanical objects and other charged particles such as electrons.
Quantum amplification of mechanical oscillator motion
S.C. Burd, R. Srinivas, J.J. Bollinger, A.C. Wilson, D.J. Wineland, D. Leibfried, D.H. Slichter and D.T.C. Allcock.
Science. Published online June 20, 2019.
Contact information: Shaun Burd shaun.burd@nist.gov Tel: (303) 497-5783 Web: Ion Storage Group
National Institute of Standards and Technology (NIST)