New study reveals the mystery behind the formation of hollowed nanoparticles during metal oxidation
- Marine Le Bouar
- May 8, 2017
- 4 min read
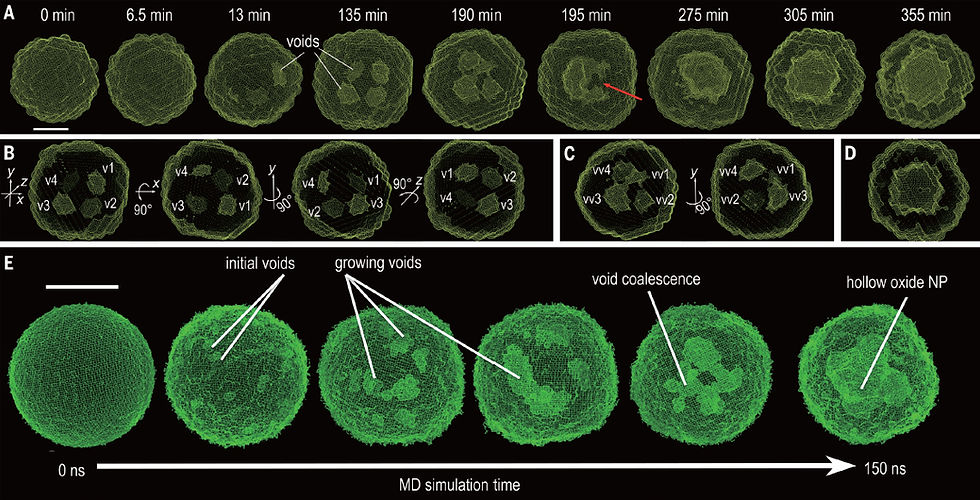
Rust usually indicates neglect; it undermines the structures and tools we rely on every day, from cars to bridges and buildings.
But if carefully controlled, the same process that creates rust – metal oxidation – could offer scientists ways to advance state-of-the-art battery or drug delivery technologies.
To achieve such control, scientists must first understand exactly how the oxidation process works. With the help of synchrotrons and supercomputers, researchers at the U.S. Department of Energy’s (DOE) Argonne National Laboratory and Temple University are illustrating the process on a finer scale than ever before.
In a paper published today in Science, Argonne and Temple University researchers describe the behavior of metal nanoparticles by watching them in real time as they oxidized. By using a combination of X-ray scattering and computational simulation, the researchers were able to observe and model the changes in nanoparticle geometry as they occurred.
This knowledge adds to our understanding of fundamental everyday reactions like corrosion, and builds a foundation for developing new techniques to image, manipulate and control such reactions at the atomic scale.
“During oxidation of metals, there is a directional flow of material across an interface which can sometimes lead to the formation of holes in the atomic lattice. This process is known as the Kirkendall effect. If well understood, it can be used to design exotic materials at the nanoscale,” said computational scientist Subramanian Sankaranarayanan, one of the principal investigators of the study and a researcher at Argonne’s Center for Nanoscale Materials.
In their study, researchers sought to understand the Kirkendall effect in small particles of iron during oxidation at the nanoscale level, specifically in the 10-nanometer range.
At this scale, roughly 10,000 times thinner than a sheet of paper, iron nanoparticles exposed to an oxygen environment exhibit a unique property – they form exotic structures, such as hollowed-out nanoparticles or nanoshells, which already have been used as electrodes in battery applications and as vehicles for drug delivery in medicine. The shape, structure and distribution of the holes in these nanoshells depend on how oxidation progresses in time.
“Transmission electron microscopy is widely used in nanomaterial research; however, conventional microscopy is incapable of investigating the structural evolution of the nanoparticles under real reaction conditions. For this reason, we used the powerful X-ray sources at the Advanced Photon Source to conduct the in situ, or ‘in place,’ characterization,” said Xiaobing Zuo, a beamline scientist at the Advanced Photon Source and one of the principal investigators in the study. “The X-rays are nondestructive to the nanoparticles and provide high-resolution 3-D structures.”
Imaging was conducted on nanoparticles that were synthesized to be of similar size and shape. “The high uniformity of the sample is another key success of this study; having particles of the same structure and size was critical to get the 3D structure,” Zuo said.
The X-rays helped the scientists monitor structural evolution as the nanoparticles oxidized. Small-angle X-ray scattering helped characterize the morphology of the particles, including the voids within, while wide-angle X-ray scattering provided information on the atomic lattice of the nanoparticles; the combination of the two enabled researchers to experimentally investigate both the metal lattice and pore structure.
With these experimental techniques, researchers could see how voids formed at a relatively high spatial resolution, but not one that reached the level of individual atoms. For this insight, researchers turned to the supercomputing resources at the Argonne Leadership Computing Facility.
Computer simulations complemented the experimental observations and enabled the researchers to simulate the oxidation of iron nanoparticles atom-by-atom – meaning researchers could visualize the formation and breakage of bonds and track the movement of individual atoms.
X-ray experiments and multimillion-atom reactive simulations were performed on a similar particle size to facilitate direct comparison of the evolving structure.
“We needed the immense computing power of the Argonne Leadership Computing Facility’s 10-petaflop supercomputer, Mira, to perform these large-scale reactive simulations,” said co-author Badri Narayanan, an Argonne staff scientist who was a postdoctoral appointee at the time of study. “The simulations provided more detailed insight into the transformation of nanoparticles into nanoshells and the atomic-scale processes that govern their evolution.”
The ability to integrate synthesis and experimental methods (X-ray imaging at the Advanced Photon Source, and nanoparticle synthesis and transmission electron microscopy at the Center for Nanoscale Materials) with computer modeling and simulation to build new knowledge is among the most valuable aspects of the study, the authors said.
“A full 3-D evolution of morphologies of nanoparticles under real reaction conditions with sub-nanometer to atomistic resolution had not been realized until this study,” said Sankaranarayanan. “This truly exemplifies how the sum can be greater than the parts – how theory and imaging together give us information that is better than what can be obtained when these methods are used independently.”
The study, titled “Quantitative 3D Evolution of Colloidal Nanoparticle Oxidation in Solution,” is published in Science.
Other authors of this study include Yugang Sun from Temple University and Sheng Peng and Ganesh Kamath with the Center for Nanoscale Materials. The research was supported by Temple University.
The work was completed using resources at the Advanced Photon Source, the Center for Nanoscale Materials and the Argonne Leadership Computing Facility, as well as the National Energy Research Scientific Computing Center at Lawrence Berkeley National Laboratory – all DOE Office of Science User Facilities.
Computing time at the Argonne Leadership Computing Facility was awarded through the Innovative and Novel Computational Impact on Theory and Experiment (INCITE) program and the ASCR Leadership Computing Challenge.