Scientists make microscopes from droplets
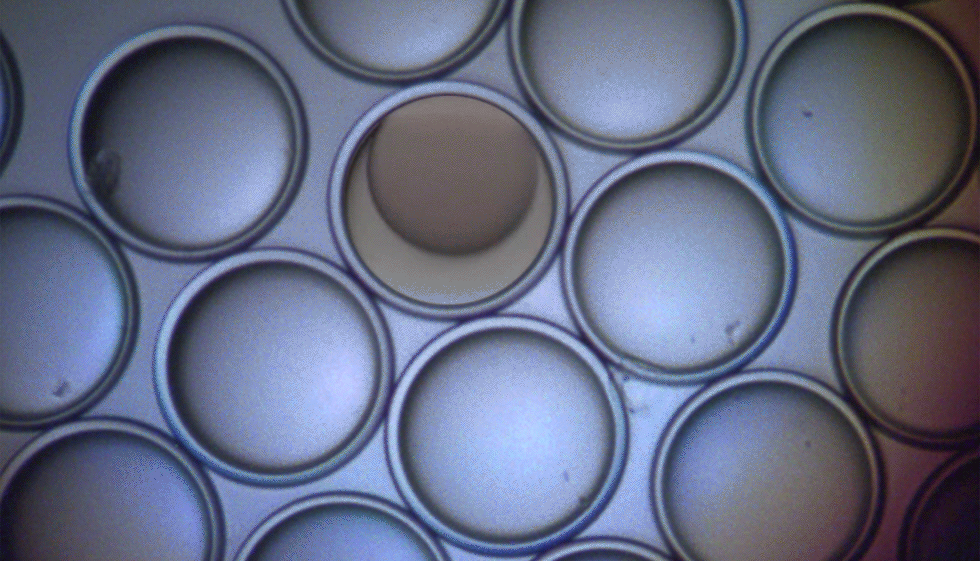
Liquid droplets are natural magnifiers. Look inside a single drop of water, and you are likely to see a reflection of the world around you, close up and distended as you’d see in a crystal ball.
Researchers at MIT have now devised tiny “microlenses” from complex liquid droplets comparable in size to the width of a human hair. They report the advance this week in the journal Nature Communications.
Each droplet consists of an emulsion, or combination of two liquids, one encapsulated in the other, similar to a bead of oil within a drop of water. Even in their simple form, these droplets can magnify and produce images of surrounding objects. But now the researchers can also reconfigure the properties of each droplet to adjust the way they filter and scatter light, similar to adjusting the focus on a microscope.
The scientists used a combination of chemistry and light to precisely shape the curvature of the interface between the internal bead and the surrounding droplet. This interface acts as a kind of internal lens, comparable to the compounded lens elements in microscopes.
“We have shown fluids are very versatile optically,” says Mathias Kolle, the Brit and Alex d'Arbeloff Career Development Assistant Professor in MIT’s Department of Mechanical Engineering. “We can create complex geometries that form lenses, and these lenses can be tuned optically. When you have a tunable microlens, you can dream up all sorts of applications.”
For instance, Kolle says, tunable microlenses might be used as liquid pixels in a three-dimensional display, directing light to precisely determined angles and projecting images that change depending on the angle from which they are observed. He also envisions pocket-sized microscopes that could take a sample of blood and pass it over an array of tiny droplets. The droplets would capture images from varying perspectives that could be used to recover a three-dimensional image of individual blood cells.
“We hope that we can use the imaging capacity of lenses on the microscale combined with the dynamically adjustable optical characteristics of complex fluid-based microlenses to do imaging in a way people have not done yet,” Kolle says.
Kolle’s MIT co-authors are graduate student and lead author Sara Nagelberg, former postdoc Lauren Zarzar, junior Natalie Nicolas, former postdoc Julia Kalow, research affiliate Vishnu Sresht, professor of chemical engineering Daniel Blankschtein, professor of mechanical engineering George Barbastathis, and John D. MacArthur Professor of Chemistry Timothy Swager. Moritz Kreysing and Kaushikaram Subramanian of the Max Planck Institute of Molecular Cell Biology and Genetics are also co-authors.
Shaping a curve
The group’s work builds on research by Swager’s team, which in 2015 reported a new way to make and reconfigure complex emulsions. In particular, the team developed a simple technique to make and control the size and configuration of double emulsions, such as water that was suspended in oil, then suspended again in water. Kolle and his colleagues used the same techniques to make their liquid lenses.
They first chose two transparent fluids, one with a higher refractive index (a property that relates to the speed at which light travels through a medium), and the other with a lower refractive index. The contrast between the two refractive indices can contribute to a droplet’s focusing power. The researchers poured the fluids into a vial, heated them to a temperature at which the fluids would mix, then added a water-surfactant solution. When the liquids were mixed rapidly, tiny emulsion droplets formed. As the mixture cooled, the fluids in each of the droplets separated, resulting in droplets within droplets.
To manipulate the droplets’ optical properties, the researchers added certain concentrations and ratios of various surfactants — chemical compounds that lower the interfacial tension between two liquids. In this case, one of the surfactants the team chose was a light-sensitive molecule. When exposed to ultraviolet light this molecule changes its shape, which modifies the tension at the droplet-water interfaces and the droplet’s focusing power. This effect can be reversed by exposure to blue light.
“We can change focal length, for example, and we can decide where an image is picked up from, or where a laser beam focuses to,” Kolle says. “In terms of light guiding, propagation, and tailoring of light flow, it’s really a good tool.”
Optics on the horizon
Kolle and his colleagues tested the properties of the microlenses through a number of experiments, including one in which they poured droplets into a shallow plate, placed under a stencil, or “photomask,” with a cutout of a smiley face. When they turned on an overhead UV lamp, the light filtered through the holes in the photomask, activating the surfactants in the droplets underneath. Those droplets, in turn, switched from their original, flat interface, to a more curved one, which strongly scattered light, thereby generating a dark pattern in the plate that resembled the photomask’s smiley face.
The researchers also describe their idea for how the microlenses might be used as pocket-sized microscopes. They propose forming a microfluidic device with a layer of microlenses, each of which could capture an image of a tiny object flowing past, such as a blood cell. Each image would be captured from a different perspective, ultimately allowing recovery of information about the object’s three-dimensional shape.
“The whole system could be the size of your phone or wallet,” Kolle says. “If you put some electronics around it, you have a microscope where you can flow blood cells or other cells through and visualize them in 3-D.”
He also envisions screens, layered with microlenses, that are designed to refract light into specific directions.
“Can we project information to one part of a crowd and different information to another part of crowd in a stadium?” Kolle says. “These kinds of optics are challenging, but possible.”