Aluminum nitride may be engineered to advance quantum computing
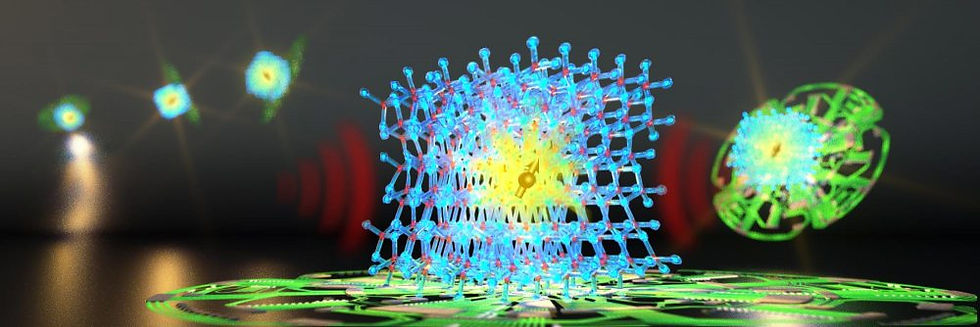
Quantum computers have the potential to break common cryptography techniques, search huge datasets and simulate quantum systems in a fraction of the time it would take today’s computers. But before this can happen, engineers need to be able to harness the properties of quantum bits, or qubits.
Currently, one of the leading methods for creating qubits in materials involves exploiting the structural atomic defects in diamond. But several researchers at the University of Chicago and Argonne National Laboratory believe that if an analogue defect could be engineered into a less expensive material, the cost of manufacturing quantum technologies could be significantly reduced. Using supercomputers at the National Energy Research Scientific Computing Center (NERSC), located at the Lawrence Berkeley National Laboratory, these researchers have identified a possible candidate in aluminum nitride. Their findings were published last February in Scientific Reports.
“Silicon semiconductors are reaching their physical limits—it’ll probably happen within the next five to 10 years—but if we can implement qubits into semiconductors, we will be able to move beyond silicon,” said Hosung Seo, UChicago postdoctoral researcher and the paper’s lead author.
“Our community has been looking at diamond for some time, but it is interesting to study a less expensive material; our motivation is to find a practical and affordable replacement for silicon in semiconductors. Aluminum nitride is a perfect candidate because it is much cheaper than diamond and there are a number of technologies that can be developed starting from aluminum nitride wafers,” said co-author Marco Govoni, postdoctoral researcher at UChicago and Argonne National Laboratory.
Seo and Govoni are members of Giulia Galli’s research group. Also a co-author on the paper, Galli is the Liew Family Professor in Electronic Structure and Simulations at the Institute for Molecular Engineering.
Strange Quantum World
Quantum mechanics describes the laws of nature on the scale of individual atoms, nuclei and electrons. At the quantum scale, physics gets strange. Take for example quantum entanglement, which occurs when pairs or groups of particles interact in such a way that the state of each particle cannot be described individually. Instead, the state must be described for the system as a whole. In other words, entangled particles act as a unit.
Another peculiar phenomenon of quantum mechanics is superposition, which occurs when two quantum states are added together to make another valid quantum state.
Whereas a conventional computer bit encodes information as either zero or one, a qubit can be zero, one, or superposition of states—both zero and one at the same time. And, if these qubits could be linked or entangled in a quantum computer, problems that cannot be solved today with conventional computers could be tackled.
Today, one of the most promising solid-state qubits is created when a nitrogen atom occupies a place near a vacant site in a diamond’s carbon lattice; this defect is called a nitrogen-vacancy center in diamond. The presence of nitrogen is actually what gives the diamond its yellowish tint.
Using NERSC’s Edison supercomputer, the researchers found that by applying strain to aluminum nitride, one could create structural defects that may be harnessed as qubits similar to the one seen in diamond. Their calculations were performed using different levels of theory and the WEST code developed at UChicago by Govoni, Galli and other researchers in the Galli group.
“The WEST code allowed us to accurately predict the position of the defect levels in the band-gap of semiconductors,” said Seo. “Ideally, we want to have defect levels in the middle of the band-gap of materials because this means that the defect’s electronic structure is well isolated from that of the host material. This is important for the qubit’s stability, to avoid de-coherence.”
Govoni said the group couldn’t have done this work without NERSC resources.
“In order to simulate these quantum defects, you also need to accurately simulate the surrounding environment—this requires a lot of computational power,” he said. “Basically you have a lot of atoms and a lot of electrons, and then in the middle of your simulation there is a defect which is the one you want to focus on, but it interacts with all the rest.”
The next step for Seo, Govoni and Galli is to work with experimenters to see if their theoretical predictions can be confirmed in a laboratory.
Reference:
“Design of defect spins in piezoelectric aluminum nitride for solid-state hybrid quantum technologies,” by Hosung Seo, Marco Govoni, and Giula Galli, Scientific Reports, published online Feb. 15, 2016, DOI:10.1038/srep20803